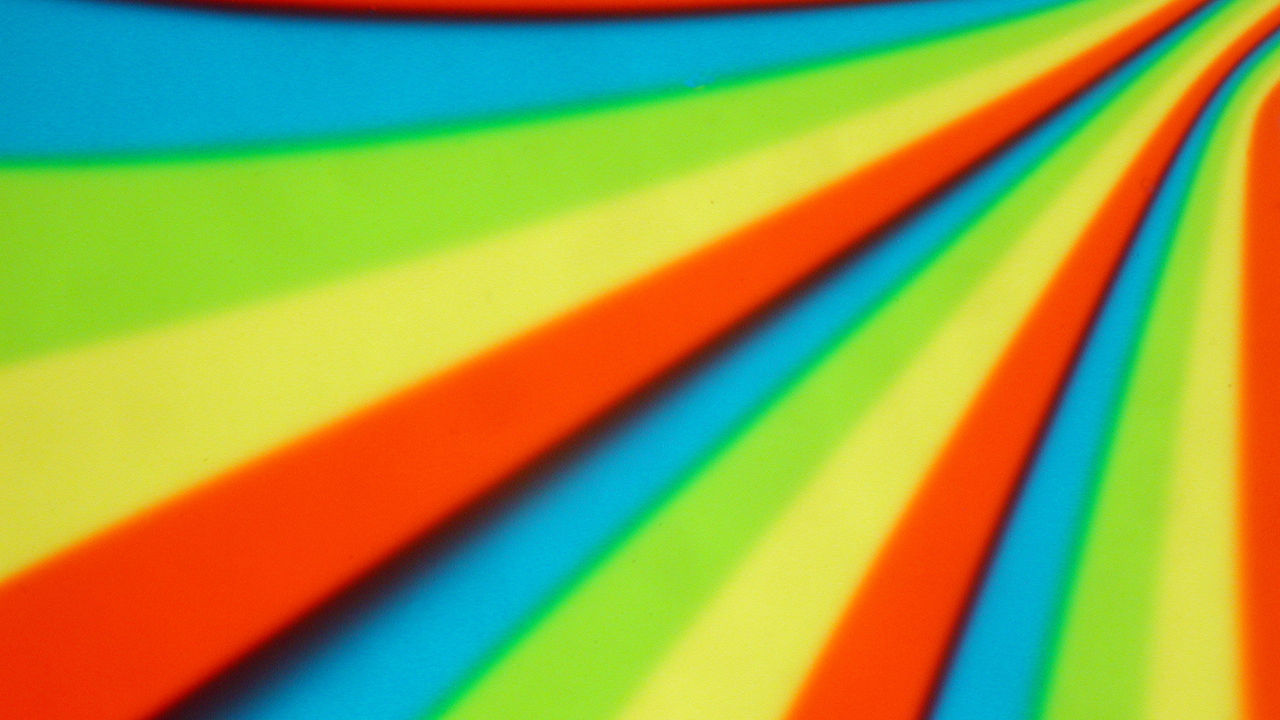

Pronounced Folk :-)
BioMedicine Projects
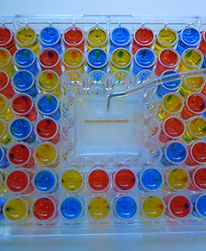
Cancer Diagnostics using Microfluidics
Tumor progression is profoundly influenced by interactions of cancer cells with their changing environment. The tumor mass consists not only of a heterogeneous population of cancer cells but also a variety of resident and infiltrating host cells, secreted factors and extracellular matrix (ECM) proteins, collectively known as the tumor microenvironment (TME). Cells and molecules of the immune system are key elements of the TME; tumors can contain both cancer-promoting as well as cancer-killing sub-types of immune inflammatory cells.
There is a lack of tools that combine deep-tissue analysis with the manipulation of live tissues on a sub-millimeter scale. Engineering, physical, and chemical approaches have been used in oncology for a long time now, including recent microfluidic approaches for drug testing submillimeter tumor pieces, organoids-on-chip, multiplexed drug testing on tumor slices, and implantable or needle microdelivery devices.
Microfluidic technology is well matched to the challenges of probing live human tumors because 1) the amount of tissue that is available from human donors is always scarce; and 2) the structural features of the TME can be highly heterogenous within a tumor, requiring microscale precision in order to properly target and understand the contributions of these unique zones to therapeutic response.
References:
L. Horowitz, A. D. Rodriguez, Allan Au-Yeung, Kevin W. Bishop, Lindsey A. Barner, Gargi Mishra, Aashik Raman, Priscilla Delgado, Jonathan T. C. Liu, Taranjit S. Gujral, Mehdi Mehrabi, Mengsu Yang, Robert H. Pierce, and A. Folch, “Microdissected “cuboids” for microfluidic drug testing of intact tissues”, Lab on a Chip 21: 122 (2021).
L.F. Horowitz, A.D. Rodriguez, Z. Dereli-Korkut, R. Lin, K. Castro, A. Mikheev, R.J. Monnat, A. Folch, and R.C. Rostomily, “Multiplexed drug testing of tumor slices using a microfluidic platform”, Nature Precision Oncology 4: 12 (2020).
A.D. Rodriguez, L.F Horowitz, K. Castro, H. Kenerson, N. Bhattacharjee, G. Gandhe, A.Raman, R.J. Monnat, R.S. Yeung, R.C. Rostomily, and A. Folch, “A Microfluidic Platform for Functional Testing of Cancer Drugs on Intact Tumor Slices”, Lab Chip 20: 1658 (2020).
L.F. Horowitz, A. D. Rodriguez, T. Ray, and A. Folch, “Microfluidics for Interrogating Live Intact Tissues”, Microsystems & Nanoengineering 6: 69 (2020).
Rumaner, M., Horowitz, L. F., Ovadya, A., & Folch, A. Thread as a Low-Cost Material for a Microfluidic Chemosensitivity Assay on Intact Tumor Slices. Micromachines 10: 481 (2019).
Tim C. Chang, Weiliang Tang, William Jen Hoe Koh, Alexander J. E. Rettie, Mary J. Emond, Raymond J. Monnat, Jr., and Albert Folch, "Microwell Arrays Reveal Cellular Heterogeneity During the Clonal Expansion of Transformed Human Cells", Technology 3: 163-171 (2015).
Tim C. Chang, Andrei M. Mikheev, Wilson Huynh, Raymond J. Monnat, Jr., Robert C. Rostomily, and Albert Folch, "Parallel Microfluidic Chemosensitivity Testing on Individual Slice Cultures", Lab on a Chip 14: 4540-4551 (2014).
Sidorova, J.M. Li, N., Schwartz, D.C., Folch, A., and Monnat Jr., R.J. “Microfluidic-assisted analysis of replicating DNA molecules”, Nature Protocols 4: 849 (2009).
Sidorova, J.M., Li, N., Folch, A., and Monnat Jr., R.J. “The RecQ helicase WRN is required for normal replication fork progression after DNA damage or replication fork arrest”, Cell Cycle 7: 796 (2008).

Axon Guidance On a Chip [ended]
During development of the nervous system the response of growing axons to their environment is critical to the formation of the complex wiring pattern between neurons. Growth and guidance factors combined with extracellular matrices influence the speed and direction of axonal growth. Although much progress has been made in identifying the factors that influence axonal growth, as well as how axons respond to these factors individually, much less is known about how axons behave in response to the combined effects of multiple factors. To fully understand how axonal growth is regulated ideally this should be studied in vivo. The ability to express fluorescent protein markers in neurons has made such an approach possible. However, it is still limited by a variety of factors. Imaging individual live axons deep in brain tissue is still restricted to layers less than 600 microns. Phototoxicity limits the time lengths of such observations. More importantly, the environment is difficult to characterize or control.
As a complementary approach, we are developing in vitro environments that potentially mimic some of the complexity found in vivo, in particular the development of the anterior visual pathway. In this system, the axon trajectories are simple, multiple relevant guidance molecules have been identified already (many tested with explants in vitro), and a common cause of blindness (Optic Nerve Hypoplasia) is associated with defects in this process. Additionally, the patterns of guidance molecules found on the flat anatomy of the retina is ideally suited to mimicking by micropatterning and microfluidics techniques. This mimicry is accomplished by combining microfluidics patterning of diffusible gradients and substrate patterning of axon pathfinding cues, including axon guidance factors and extracellular matrix molecules. As a source of highly homogeneous cell populations, we use isolated mouse retinal ganglion cells (RGCs), a cell type that responds to Netrin-1 gradients. For experiments designed to maximize the integrity of the cells (isolation procedures are damaging to cells), we will use retinal explants and we microfluidically isolate the axons from their somas. RGCs (or their axons) are exposed to various soluble factors that have previously been shown to affect their axon growth in vivo. Combinatorial microfluidic mixers allow us to test the effects of multiple factors on the direction and speed of axonal growth of RGCs. These experiments allow us to quantitatively examine the basic principles that govern axon pathfinding in the development of the anterior visual pathway and help to better understand the basis of developmental defects in axon growth that alter the organization and function of the nervous system.
References:
Bhattacharjee, N. and Folch, A. "Large-scale microfluidic gradient arrays reveal axon guidance behaviors in hippocampal neurons", Microsystems & Nanoengineering 3: 1-14 (2017).
Bhattacharjee, N., Li, N., Keenan, T.M., and Folch, A. “A Neuron-Benign Microfluidic Gradient Generator for Studying the Growth of Mammalian Neurons towards Axon Guidance Factors”, Integrative Biology 2: 669 (2010).
Keenan, T.M. and Folch, A. “Biomolecular gradients in cell culture systems”, Lab Chip 8: 34 (2008).
Keenan, T.M., Hsu, C.-H., and Folch, A. “Microfluidic “Jets” for Generating Steady-State Gradients of Soluble Molecules on Open Surfaces”, Appl. Phys. Lett. 89: 114103 (2006).
Keenan, T.M., Hooker, A., Spilker, M. E., Boggy, G. J., Li, N., Vicini, P., and Folch, A. “Automated Identification of Axonal Growth Cones in Time-Lapse Image Sequences”, J. Neurosci. Methods 151: 232 (2006).
Li, N. and Folch, A. “Integration of topographical and biochemical cues by axons during growth on microfabricated 3-D substrates”, Experimental Cell Research 311: 307 (2005).