top of page
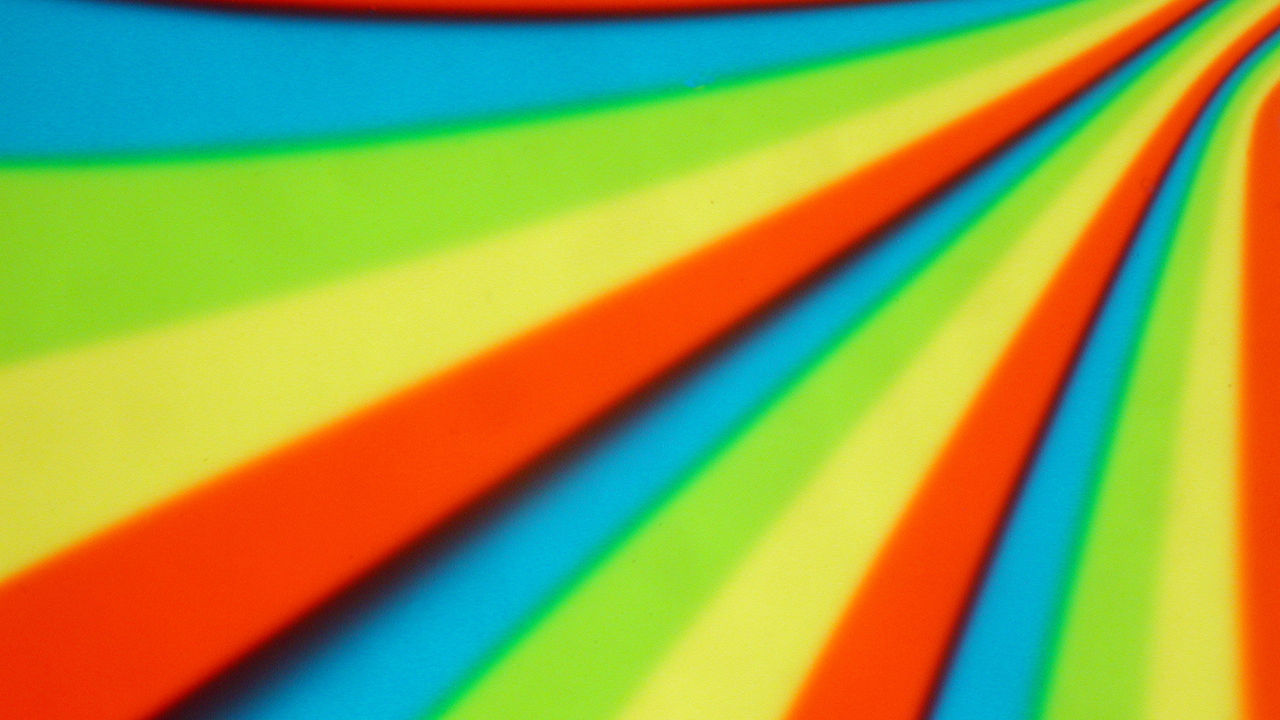

Pronounced Folk :-)
Folch Lab Publications
Please click on the numbers to download the PDFs (most of them free)
82 Alireza Ahmadianyazdi, Isaach J. Miller, and A. Folch. “Tunable resins with PDMS-like elastic modulus for stereolithographic 3D-printing of multimaterial actuators”, Lab Chip, 23: 4019 (2023).
We introduce low-viscous photopolymers based on a tunable blend of the monomers poly(ethylene glycol) diacrylate (PEGDA, Mw ∼ 258) and the monoacrylate poly(ethylene glycol methyl ether) methacrylate (PEGMEMA, Mw ∼ 300). Tuning the PEGMEMA content from 0% to 40% (v/v) alters the elastic modulus of the printed plastics by ∼400-fold, reaching that of PDMS.
81 Y. T. Kim, Alireza Ahmadianyazdi, and A. Folch. “A 'print-pause-print' protocol for 3D printing microfluidics using multimaterial stereolithography”, Nature Protocols, 18: 1243 (2023). We describe a simple protocol for exchanging the resin in the vat during stereolithography, which allows for building microfluidic devices featuring more than one material without alignment methods.
80 G. Spennati, L. F. Horowitz, D. J. McGarry, D. A. Rudzka, G. Armstrong, M. F. Olson, A. Folch, and H. Yin. “Organotypic platform for studying cancer cell metastasis”, Experimental Cell Research, 401: 112527 (2021). This paper evaluates the use of organotypic liver and brain slices to study cancer metastasis and illustrates the potential for organotypic tissue slices to more closely mimic in vivo conditions during cancer cell metastasis than most in vitro models.
79 L. Horowitz, A. D. Rodriguez, Allan Au-Yeung, Kevin W. Bishop, Lindsey A. Barner, Gargi Mishra, Aashik Raman, Priscilla Delgado, Jonathan T. C. Liu, Taranjit S. Gujral, Mehdi Mehrabi, Mengsu Yang, Robert H. Pierce, and A. Folch, “Microdissected “cuboids” for microfluidic drug testing of intact tissues”, Lab on a Chip, 21: 122 (2021).
This paper describes the microdissection of live biopsies from tumors and healthy tissues into large numbers of regular, cuboidal-shaped intact pieces that can be individually trapped in a multiwell-format microfluidic device for selective drug treatment. --> Featured in Fred Hutch's Science SpotLight
78 L. Horowitz, A. D. Rodriguez, T. Ray, and A. Folch, “Microfluidics for Interrogating Live Intact Tissues”, Microsystems & Nanoengineering, 6: 69 (2020).
This broad review covers all microfluidic devices that have been used to address the biology or pharmacology of intact tissues (i.e. without dissociation), including implantable or skin-based microfluidic systems and those used to probe small animals and plant tissues.
77 A.D. Rodriguez, L.F Horowitz, K. Castro, H. Kenerson, N. Bhattacharjee, G. Gandhe, A.Raman, R.J. Monnat, R.S. Yeung, R.C. Rostomily, and A. Folch, “A Microfluidic Platform for Functional Testing of Cancer Drugs on Intact Tumor Slices”, Lab Chip, 20: 1658 (2020).
Here we describe the fabrication process of our platform in detail, we characterize the fluidic performance of the device, and demonstrate on-device drug-response testing with tumor slices from xenografts and from a patient colorectal tumor.
76 L.F. Horowitz, A.D. Rodriguez, Z. Dereli-Korkut, R. Lin, K. Castro, A. Mikheev, R.J. Monnat, A. Folch, and R.C. Rostomily, “Multiplexed drug testing of tumor slices using a microfluidic platform”, Nature Precision Oncology, 4: 12 (2020).
We developed a digitally-manufactured microfluidic platform for multiplexed drug testing of intact cancer slice cultures, and demonstrate the use of this platform to evaluate drug responses in slice cultures from human glioma xenografts and patient tumor biopsies.
75 J.W. Cheng, C.G. Sip, P.R. Lindstedt, R. Boitano, B.M. Bluestein, L.J. Gamble, and A. Folch, “'Chip-on-a-Transwell' Devices for User-Friendly Control of the Microenvironment of Cultured Cells”, ACS Applied Bio Materials 2: 4998 (2019).
We develop a technique for micropatterning cells inside a Transwell as well as for patterning small apertures in the Transwell membrane as a tool to engineer the environment of cultured cells.
74 A. Kuo, N. Bhattacharjee, Y.-S. Lee, K. Castro, Y. T. Kim, and A. Folch, “High-Precision Stereolithography of Biomicrofluidic Devices”, Advanced Materials Technologies, 1800395 (2019).
We added the photosensitizer isopropyl thioxanthone to achieve higher absorbance (and thus resolution) using desktop 3D-printers without compromising transparency or biocompatibility.
73 M. Rumaner, L. Horowitz, A. Ovadya, and A. Folch, “Thread as a low-cost material for microfluidic assays on intact tumor slices”, Micromachines 10: 481 (2019).
We explored the use of threads such as cotton to inexpensively deliver cancer drugs to selected locations of tumor slices.
72 A. Naderi, N. Bhattacharjee, and A. Folch, “Digital Manufacturing for Microfluidics”, Annual Review of Biomedical Engineering 21: 325 (2019).
A review of microfluidic systems fabricated by digital manufacturing technologies, including 3D-printing, laser-cutting and CNC milling.
71 YT Kim, S. Bohjanen, N. Bhattacharjee, and A. Folch, “Partitioning of hydrogels in 3D-printed microchannels”, Lab on a Chip 19: 3086 (2019).
We present 3D-printable microfluidic designs for trapping hydrogels so they can be used as porous barriers in microfluidic devices.
70 V. Balaji, K. Castro, and A. Folch, “A Laser-Engraving Technique for Portable Micropneumatic Oscillators”, Micromachines 9, 426 (2018).
We present microfluidic logic elements (in particular, a self-driven pneumatic ring oscillator) that can be manufactured in plastic by laser cutting.
69 N. Bhattacharjee, C. Parra‐Cabrera, Y. T. Kim, A. P. Kuo, and A. Folch, “Desktop‐Stereolithography 3D‐Printing of a Poly (dimethylsiloxane)‐Based Material with Sylgard‐184 Properties”, Advanced Materials 30, 1800001 (2018).
We present a novel PDMS resin formulation compatible with desktop SLA printers.
68 Y. T. Kim, K. Castro, N. Bhattacharjee, and A. Folch, “Digital Manufacturing of Selective Porous Barriers in Microchannels Using Multi-Material Stereolithography”, Micromachines 9, 125 (2018).
We demonstrate a method for co-printing PEG-DA hydrogels (MW ~ 700) within microfluidic devices printed in low-MW PEG-DA (MW ~ 258). The method allows for the assembly-free manufacturing of porous barriers within biomicrofluidic devices.
67 Y. S. Lee, N. Bhattacharjee, and A. Folch, “3D-Printed Quake-Style Microvalves and Micropumps”, Lab on a Chip 18, 1207 (2018).
We demonstrate the stereolithographic printing of a modified Quake valve/pump design in a transparent, PEG-DA-based biocompatible resin.
66 N. Bhattacharjee and A. Folch, “Large-Scale Microfluidic Gradient Arrays Reveal Axon Guidance Behaviors in Hippocampal Neurons”, Microsystems & Nanoengineering 3, 17003 (2017).
This is the first report of a large-scale array (1024 units) of single-cell microfluidic gradient generators operating in parallel. We used the array to elicit axon guidance growth of single (mouse) hippocampal neurons. We observed that neurons exposed to low netrin concentrations were repelled by netrin gradients whereas neurons exposed to high netrin concentrations were attracted by the same gradients, among other observations.
65 A. Rountree, A. Karkamkar, G. Khalil, A. Folch, D. L. Cook, and I. R. Sweet, “BaroFuse, a novel pressure-driven, adjustable-throughput perfusion system for tissue maintenance and assessment”, Heliyon 2, e00210 (2016).
We present a transparent perfusion system 3D-printed in the commercial resin Watershed for the study of intact tissue (liver slices and pancreatic islets).
64 N. Bhattacharjee, L. F. Horowitz, and A. Folch, “Continuous-flow multi-pulse electroporation at low DC voltages by microfluidic flipping of the voltage space topology”, Applied Physics Letters 109, 163702 (2016).
We present a novel design for microscale, multi-pulse electroporation that bypasses the use of complex electronics to create the voltage pulses and uses only a few tens of volts DC, resulting in high efficiency and viability on several cell types.
63 A. Urrios, C. Parra-Cabrera, A M. Gonzalez-Suarez, N. Bhattacharjee, L. G. Rigat-Brugarolas, U. Nallapatti, J. Samitier, C. A. DeForest, F. Posas, J. L. Garcia-Cordero, and A. Folch, “3D-Printing of transparent bio-microfluidic devices in PEG-DA”, Lab on a Chip 16: 2287 (2016).
The first report of stereolithographically-printed microfluidic devices in fully transparent PEG-DA (MW ~ 258), a biocompatible polymer that was shown to support mammalian cell culture.
62 N. Bhattacharjee, A. Urrios, Shawn Kang, and A. Folch, “The upcoming 3D-printing revolution in microfluidics”, Lab on a Chip 16: 1720 (2016).
Critical review of 3D-Printing with an emphasis on microfluidics. We provide an overview of the various 3D-Printing techniques used to date to fabricate microfluidic systems and the critical barriers that 3D-Printing needs to overcome compared to molding technologies. --> Cover
61 J. Cheng, T. Chang, N. Bhattacharjee, and A. Folch, “An open-chamber flow-focusing device for focal stimulation of micropatterned cells”, Biomicrofluidics 10, 024122 (2016).
We present an open-chamber microfluidic device that delivers hydrodynamically focused streams of soluble reagents to cells over several hours. We demonstrate the differentiation of myoblasts into myotubes, a process that occurs in 7–10 days and is difficult to achieve in closed chamber microfluidic devices.
60 A.K. Yetisen, A.F. Coskun, G. England, S. Cho, H. Butt, J. Hurwitz, M. Kolle, A. Khademhosseinni, A.J. Hart, A. Folch, and S.H. Yun, "Art on the Nanoscale and Beyond", Advanced Materials 28, 1713 (2016).
Nano-/microscale art practices creative activity at small dimensions. It brings together expertise in art and sciences to spur creativity, promote scientific advances, and challenge dominant paradigms. Miniaturized artworks in semiconductors, microfluidics, and nanostructures are reviewed. The perceived limitations of nano-/microscale artistic practice are discussed and potential future directions are highlighted. --> Cover
59 A.K. Au, N. Bhattacharjee, L. Horowitz, T. Chang, and A. Folch, "3D-printed microfluidics", Angewandte Chemie 55, 3862 (2016).
We provide an overview of the various 3D-printing processes and a comparison with soft lithography.
58 W. Lee, D. Kwon, W. Choi, G.Y. Jung, A. Au, A. Folch, and S. Jeon, "3D-printed microfluidic device for the detection of pathogenic bacteria using size-based separation in helical channel with trapezoid cross-section", Scientific Reports 5, 7717 (2015).
We report a 3D-printed helical microchannel device with trapezoid cross-section that is used to detect pathogenic bacteria using magnetic nanoparticle clusters (MNCs).
57 T. Chang, W. Tang, W.J.H. Koh, M. Emond, R.J. Monnat Jr., and A. Folch, “Microwell arrays reveal cellular heterogeneity during the clonal expansion of transformed human cells”, Technology 3, 163 (2015).
We demonstrate the utility of PDMS microwell arrays by quantifying the proliferation and senescence of isogenic cells which expressed or had been depleted of the human Werner syndrome protein.
56 A. Au, N. Bhattacharjee, L.F. Horowitz, T.C. Chang, and A. Folch, “3D-printed microfluidic automation”, Lab Chip 15, 1934 (2015).
We present the first 3D-printable valves, pumps and fluidic switches. --> Cited in Lab on a Chip Highlights & Named in Lab on a Chip Most Accessed Articles (Top 30 Collection) of 2015
55 T. Chang, A.M. Mikheev, W. Huynh, R.J. Monnat Jr., R.C. Rostomily, and A. Folch, “Parallel microfluidic chemosensitivity testing on individual slice cultures”, Lab Chip 14, 4540 (2014).
A user-friendly device based on a 96-well platform allows for testing multiple chemotherapy compounds simultaneously on organotypic cultures of brain slices.
54 W. Lee, D. Kwon, B. Chung, G.Y. Jung, A. Au, A. Folch, and S. Jeon, "Ultrarapid detection of pathogenic bacteria using a 3D immunomagnetic flow assay", Anal. Chem. 86, 6683 (2014).
We developed a 3D-printed cylindrical microchannel for the ultrarapid immunocapture of bacteria with antibody-functionalized magnetic nanoparticles.
53 A. C. Ventura, A. Bush, G. Vasen, M. A. Goldín, B. Burkinshaw, N. Bhattacharjee, A. Folch, R. Brent, A. Chernomoretz, and A. Colman-Lerner, “Utilization of extracellular information before ligand-receptor binding reaches equilibrium expands and shifts the input dynamic range”, Proceedings of the National Academy of Sciences 111, E3860 (2014).
We produce chemical gradients in yeast with our microjets gradient generators to understand how cells use “systems-level” mechanisms to process complex environmental information.
52 C. Sip and A. Folch, "Stable chemical bonding of porous membranes and poly(dimethyl siloxane) devices for long-term cell culture", Biomicrofluidics 8, 036504 (2014).
We present a technique for the robust bonding of porous membranes to PDMS devices for long-term use in combination with cell culture fluids.
51 A.K. Au, W. Lee, and A. Folch, "Mail-order microfluidics: evaluation of stereolithography for the production of microfluidic devices", Lab on a Chip 14, 1294 (2014).
We explore the 3D capabilities, resolution, and optical clarity of microfluidic devices fabricated by stereolithography using a mail-order service, and we compare the cost and prototyping speed of soft lithography with those of stereolithography.
50 C.R. Easton, K. Weir, A. Scott, S.P. Moen, Z. Barger, A. Folch, R. Hevner, and W.J. Moody, "Genetic elimination of GABAergic neurotransmission reveals two distinct pacemakers for spontaneous waves of activity in the developing mouse cortex", J. Neuroscience 34, 3854 (2014).
Using our patch clamp chip we show that genetic knockout of GAD67 (the major synthetic enzyme for GABA) selectively eliminates the picrotoxin-sensitive fraction of waves of electrical activity that are essential to CNS development.
49 C.G. Sip, N. Bhattacharjee, and A. Folch, "Microfluidic transwell inserts for generation of tissue culture-friendly gradients in well plates", Lab on a Chip 14, 302 (2014).
We present a user-friendly microfluidic gradient generator based on modified Transwell inserts that is compatible with standard tissue culture wells.
48 K.W. Moyes, C.G. Sip, W. Obenza, E. Yang, C. Horst, R.E. Welikson, S.D. Hauschka, A. Folch, and M. Laflamme, "Human embryonic stem cell-derived cardiomyocytes migrate in response to gradients of fibronectin and Wnt5a", Stem Cells and Development 22, 1 (2013).
Human embryonic stem cell-derived cardiomyocytes show robust promigratory responses to microfluidic gradients of fibronectin and Wnt5a.
47 A. Scott, A. K. Au, E. Vinckenbosch, and A. Folch, "A microfluidic D-subminiature connector", Lab on a Chip 13, 2036 (2013).
We present a novel microfluidic connector based on standard electronic components that are available worldwide.
46 P. Skafte-Pedersen, C. G. Sip, A. Folch, and M. Dufva, "Modular microfluidic systems using reversibly attached PDMS fluid control modules", Journal of Micromech. Microeng. 23, 055011 (2013).
We demonstrate the integration of PDMS-based fluid control modules with hard polymer chips made of PMMA.
45 A. Scott, K. Weir, C. Easton, W. Huynh, W. J. Moody, and A. Folch, "A microfluidic microelectrode array for simultaneous electrophysiology, chemical stimulation, and imaging of brain slices", Lab Chip 13, 527 (2013).
We demonstrate electrophysiological recordings from the surface of brain slices using a PDMS device featuring multiple apertures that function as extracellular electrodes as well as chemical stimulation points.
44 A. K. Au, H. Lai, B. R. Utela, and A. Folch, “Microvalves and Micropumps for BioMEMS”, Micromachines 2, 179 (2011).
An in-depth review of the designs of micropumps and microvalves that have been used in the BioMEMS literature.
43 C. G. Sip, N. Bhattacharjee, and A. Folch, “A Modular Cell Culture Device for Generating Arrays of Gradients Using Stacked Microfluidic Flows”, Biomicrofluidics 5, 022210 (2011).
This device reports a microfluidic gradient generator for cell culture applications based on the use of stacked laminar flows.
42 Hoyin Lai and Albert Folch, "Design and characterization of "single-stroke" peristaltic PDMS micropumps", Lab Chip 11, 336 (2011).
We demonstrate a new design of PDMS peristaltic pumps operated with a single control line.
41 Anna Boardman, Tim Chang, Albert Folch, and Norman J. Dovichi, "Indium-Tin Oxide Coated Microfabricated Device for the Injection of a Single Cell into a Fused Silica Capillary for Chemical Cytometry", Analytical Chemistry 82, 9959 (2010).
We describe a microfabricated device for the capture and injection of a single mammalian cell into a fused silica capillary for subsequent analysis by chemical cytometry.
40 Nirveek Bhattacharjee, Nianzhen Li, Thomas M. Keenan, and Albert Folch, "A neuron-benign microfluidic gradient generator for studying the response of mammalian neurons towards axon guidance factors", Integrative Biology 2, 669 (2010).
We record axonal growth of mouse embryonic cortical neurons in response to netrin gradients generated with a low-shear, open-bath microfluidic device.
39 David M. Cate, Christopher Sip, and Albert Folch, "A microfluidic platform for generation of sharp gradients in open-access culture", Biomicrofluidics 4, 044105 (2010).
We demonstrate a membrane-based gradient generator that is compatible with open cell cultures.
38 John M. Hoffman, Mitsuhiro Ebara, James J. Lai, Allan S. Hoffman, Albert Folch, and Patrick Stayton, "A helical flow, circular microreactor for separating and enriching 'smart' polymer-antibody capture reagents", Lab Chip 10, 3130 (2010).
We report a mechanistic study of how flow and recirculation in a microreactor can be used to optimize the capture and release of stimuli-responsive polymer–protein reagents on stimuli-responsive polymer-grafted channel surfaces.
37 Ellen Tenstad, Anna Tourovskaia, Albert Folch, Ola Myklebost, and Edith Rian, "Extensive adipogenic and osteogenic differentiation of patterned human mesenchymal stem cells in a microfluidic device", Lab Chip 10, 1401 (2010) --> Inside cover article.
Adipogenic and osteogenic differentiation of patterned human mesenchymal stem cells is demonstrated using long-term microfluidic perfusion.
36 Figueroa, X.A., Cooksey, G.A., Votaw, S.V., Horowitz, L.F., and Folch, A., "Large-scale investigation of the olfactory receptor space using a microfluidic microwell array", Lab Chip 10, 1120 (2010). --> Cover article & Cited in Chemical Technology Highlights section.
We show simultaneous calcium recordings of mouse dissociated olfactory sensory neurons in large microarrays so that the whole repertoire of mouse olfactory receptors is probed in one experiment.
35 Keenan, T.M., Frevert, C.W., Wu, A., Wong, V., and Folch, A., "A New Method for Studying Gradient-Induced Neutrophil Desensitization Based on an Open Microfluidic Chamber", Lab Chip 10, 116 (2010).
This paper demonstrates neutrophil chemotaxis measurements in an open microfluidic chamber.
34 Sidorova, J.M. Li, N., Schwartz, D.C., Folch, A., and Monnat Jr., R.J. "Microfluidic-assisted analysis of replicating DNA molecules", Nature Protocols 4, 849 (2009).
This paper presents detailed protocols on how to stretch DNA on glass surfaces using microfluidic channels.
33 Cooksey, G.A., Sip, C.G., and Folch, A., "A multi-purpose microfluidic perfusion system with combinatorial choice of inputs, mixtures, gradient patterns, and flow rates", Lab on a Chip 9, 417 (2009).
We demonstrate a microfluidic perfusion system where the user can choose one of 64 combinations of 2,4, 8 or 16 inlets, stepwise or smoothened gradients, homogeneized mixtures, and 16 levels of flow rates going into a chamber designed for cell culture applications.
32 Tourovskaia, A., Li, N., and Folch, A., "Localized acetylcholine receptor clustering dynamics in response to microfluidic focal stimulation with agrin", Biophys. J. 95, 3009 (2008) --> Cited in Lab on a Chip Research Highlights section.
In this paper we show that the synaptogenic factor agrin can have an important role in stabilizing agrin-predating acetylcholine receptor clusters.
31 Sidorova, J.M., Li, N., Folch, A., and Monnat Jr., R. "The RecQ helicase WRN is required for normal replication fork progression after DNA damage or replication fork arrest", Cell Cycle 7, 796 (2008).
We use flow in microchannels to stretch DNA on the surface for fundamental studies of genomic stability and prediction of DNA-targeting chemotherapy outcomes.
30 Keenan, T.M. and Folch, A. "Biomolecular gradients in cell culture systems", Lab on a Chip 8, 35 (2008) --> Cited in the cover.
In this paper we review the major biological phenomena in which biomolecule gradients are employed, traditional in vitro gradient-generating methods developed over the past 50 years, and new microfluidic devices for generating gradients.
29 Chen, H.-H., Shen, H., Heimfeld, S., Tran, K.K., Reems, J., Folch, A., and Gao, D. “A microfluidic study of mouse dendritic cell membrane transport properties of water and cryoprotectants”, Int. J. Heat and Mass Transfer 51: 5687 (2008).
28 Folch, A., "BioMEMS and cellular biology: perspectives and applications", J. Vis. Exp. 300 (2007). --> See free link.
27 Li, N., Sip, C., and Folch, A., "Microfluidic chips controlled with elastomeric microvalve arrays", J. Vis. Exp. 296 (2007). --> See free link.
26 Chen, H.-H., Purtteman, J.J.P., Heimfeld, S., Folch, A., and Gao, D. "Development of a Microfluidic Device for Determination of Cell Osmotic Behavior and Membrane Transport Properties", Cryobiol. 55, 200 (2007).
We demonstrate the measurement of volumetric changes of single cells trapped in microchannels in response to osmolarity changes for cryobiology applications.
25 Seng, K.-Y., Figueroa-Masot, X., Folch, A., and Vicini, P. "Objective Quantification of Acetylcholine Receptor Aggregation Using Fast Fourier Transforms", Comp. Meth. Prog. Biomed. 85, 220 (2007).
We were able to automate the measurement of acetylcholine receptor clustering in myotube cultures.
24 Lam, E.W., Cooksey, G.A., Finlayson, B.A., and Folch, A., "Microfluidic Circuits with Tunable Flow Resistances", Applied Physics Letters 89, 164105 (2006) --> featured in the Virtual Journal of Nanoscale Science & Technology (Vol. 14, Iss. 18).
In this paper we present the concept and implementation of a microfluidic "resistor" (a segment of a microchannel with user-controlled flow resistance).
23 Hsu, C.-H. and Folch, A., "Spatiotemporally-Complex Concentration Profiles Using a Tunable Chaotic Micromixer", Applied Physics Letters 89, 144102 (2006) --> featured in the Virtual Journal of Nanoscale Science & Technology (Vol. 14, Iss. 16).
We demonstrate the generation of temporal sequences of complex concentration profiles using a single device containing tunable microtopographies.
22 Chen, C. and Folch, A., "A High-Performance Elastomeric Patch Clamp Chip", Lab on a Chip 6, 1338 (2006).
We report a microfluidic patch clamp chip that allows for performing whole-cell recordings with unprecedented yields and exchanges of the extracellular and intracellular solutions.
21 Tourovskaia, A., Figueroa-Masot, X. and Folch, A., "Long-term Microfluidic Cultures of Myotube Microarrays for High-Throughput Focal Stimulation ", Nature Protocols 1, 1092 (2006).
In this paper we report the precise protocols for culturing and focally stimulating single-myotube microarrays in a microfluidic device.
20 Keenan, T.M., Hsu, C.-H., and Folch, A., "Microfluidic "Jets" for Generating Steady-State Gradients of Soluble Molecules on Open Surfaces", Applied Physics Letters 89, 114103 (2006) --> featured in the Virtual Journal of Nanoscale Science & Technology (Vol. 14, Iss. 13) and in the Virtual Journal of Biological Physics Research (Vol. 12, Iss. 6).
In this paper we demonstrate the use of microfluidic "jets" to create dynamic gradients of soluble molecules on open surfaces.
19 Frevert, C.W., Boggy, G., Keenan, T.M., and Folch, A., "Measurement of Cell Migration in Response to an Evolving Radial Chemokine Gradient Triggered by a Microvalve", Lab on a Chip 6, 849 (2006) --> cited in the cover.
In this paper we use a microfluidic valve to create chemotactic gradients and obtain neutrophil migration measurements correlated with spatiotemporal gradient values.
18 Tourovskaia, A., T.F. Kosar, and Folch, A., "Local Induction of Acetylcholine Receptor Clustering in Myotube Cultures Using Microfluidic Application of Agrin", Biophysical Journal 90, 2192 (2006).
This paper shows the local clustering of acetylcholine receptors on selected areas of cultured myotubes using microfluidic focal application of agrin.
17 T.F. Kosar, Tourovskaia, A., Figueroa-Masot, X., Adams, M., and Folch, A., "A Nanofabricated Planar Aperture as a Mimic of the Nerve-Muscle Contact During Synaptogenesis", Lab Chip 6, 632 (2006). --> featured in Chemical Biology (top-viewed article in May 2006) and in the Faculty of 1000 Biology.
This article demonstrates that nanofluidic delivery of soluble agrin to myotubes induces local clustering of acetylcholine receptors.
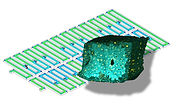
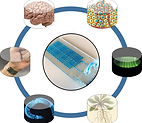
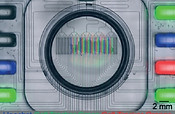


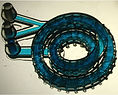


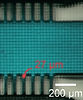
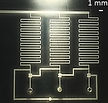

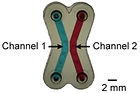
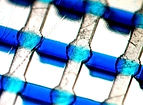

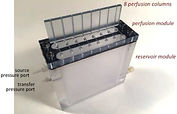
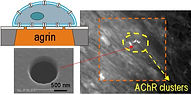
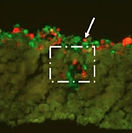

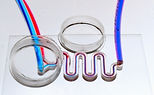
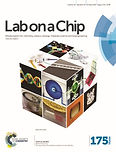



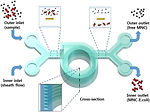

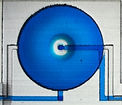
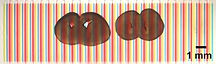

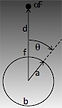

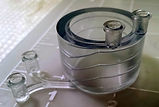
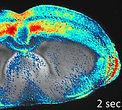
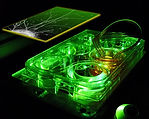
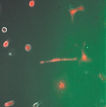
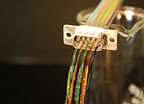

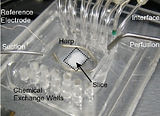
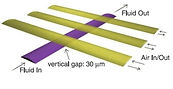
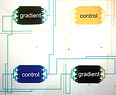
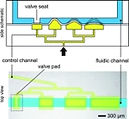

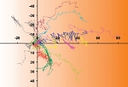
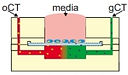
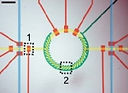
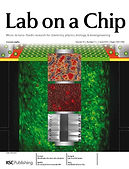
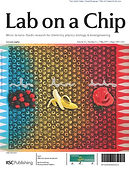



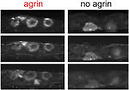


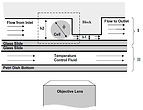
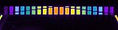
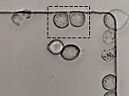


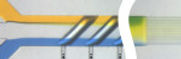
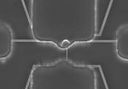
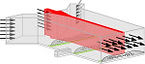
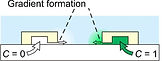
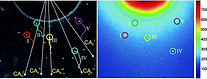
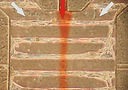
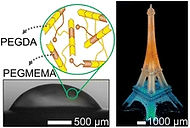
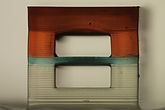
16 Keenan, T.M., Hooker, A., Spilker, M. E., Boggy, G. J., Li, N., Vicini, P., and Folch, A., "Automated Identification of Axonal Growth Cones in Time-Lapse Image Sequences", J. Neurosci. Methods 151, 232 (2006).
This paper reports an image recognition software to track and quantitate the growth of axons from sequences of time-lapse phase-contrast micrographs of neurons growing on a flat substrate.
15 Li, N. and Folch, A., "Integration of topographical and biochemical cues by axons during growth on microfabricated 3-D substrates", Experimental Cell Research 311, 307 (2005).
This paper reports effects of the microscale substrate composition and topography on axon growth in cultured embryonic cortical neurons.
14 Li, N., Hsu, C.-H., and Folch, A., "Parallel mixing of Photolithographically-Defined Nanoliter Volumes Using Elastomeric Microvalve Arrays", Electrophoresis 26, 3758 (2005).
This article demonstrates the use of microvalve arrays to create multiple titrations simultaneously (demonstration of a calcium-sensitive dye calibration).
13 Stucky, N.L., Chen, C., Kosar, T.F., and Folch, A., "Fabrication of Microfluidically-Accessible Planar Nanoholes on Elastomeric Substrates", Journal of Biomedical Nanotechnology 1, 384 (2005).
This paper reports the fabrication of nanoholes on PDMS surfaces to stimulate cells from underneath the cell culture surface.
12 Kosar, T.F., Chen, C., Stucky, N.L., and Folch, A., "Arrays of Microfluidically-Addressable Nanoholes", Journal of Biomedical Nanotechnology 1, 161 (2005).
This paper reports the fabrication of nanoholes on silicon nitride membranes to stimulate cells from underneath the cell culture surface.
11 Beebe, D. and Folch, A., “The science and applications of cell biology in microsystems”, Lab Chip 5: 10 (2005).
Short review paper.
10 Rettig, J.R. and Folch, A., "Large-Scale Single-Cell Trapping and Imaging Using Microwell Arrays", Analytical Chemistry 77, 5628 (2005).
This article demonstrates the use of microwells to trap single cells in large arrays. --> an image from this highly cited paper was used to illustrate the cover of a special issue of Lab on a Chip.
9 Hsu, C.-H., Chen, C., and Folch, A., "Microfluidic Devices with Tunable Microtopographies", Applied Physics Letters 86, 023508 (2005) --> featured in the Virtual Journal of Nanoscale Science & Technology (Vol. 11, Iss. 2) and in the Virtual Journal of Biological Physics Research (Vol. 9, Iss. 2).
This paper implements our tunable-microtopography technique inside a microfluidic channel to demonstrate novel micromixers and fluid traps.
8 Tourovskaia, A., Figueroa-Masot, X. and Folch, A., "Differentiation-on-a-chip: A Microfluidic Platform for Long-Term Cell Culture Studies", Lab on a Chip 5, 14 (2005) --> cited in the cover and featured in Sample Content (thus available free of charge).
This paper (cited almost 300 times) demonstrates the first 2-week-long cell culture in a microfluidic environment. Optionally, the cells could be selectively stimulated using heterogeneous laminar streams.
7 Hoffman, J., Shao, J., Hsu, C.-H., and Folch, A., "Elastomeric Molds with Tunable Microtopographies", Advanced Materials 16, 2201 (2004).
This paper reports a micromolding method based on molds whose microtopography can be tuned by the user, thereby producing features that are difficult or impossible to obtain by traditional photolithography.
6 Hsu, C.-H., Chen, C., and Folch, A., "'Microcanals' for Micropipette Access to Single Cells in Microfluidic Environments", Lab on a Chip 4, 420 (2004) --> featured in the RSC's Chemical Biology Virtual Journal (2004, Iss. 19).
This paper reports the first implementation of open-air microfluidic channels and their use to probe single cells with micropipettes within a microfluidic environment.
5 Neils, C. M., Tyree, Z., Finlayson, B., and Folch, A., "Combinatorial Mixing of Microfluidic Streams", Lab on a Chip 4, 342 (2004) --> featured in The Washington Post.
In this paper we describe a microfluidic mixer that outputs all the sixteen combinations of four titrations of two dyes in continuous flow.
4 Li, N., Tourovskaia, A., and Folch, A., "Biology on a Chip: Microfabrication in Cell Culture Studies", Critical Reviews in Biomedical Engineering 31, 68 (2003).
This paper reviews work on cell culture studies that incorporate microfabrication in order to modulate the microenvironment of cells.
3 Chen, C., Hirdes, D., and Folch, A., "Gray-Scale Photolithography Using Microfluidic Photomasks", Proceedings of National Academy of Sciences 100, 1499 (2003) --> featured in the New York Times, Materials Today, Science News, Physics World, Photonics Spectra, Biophotonics International, and Physics Web.
This paper reports the development of photolithographic masks that contain fluidic features of tunable opacity, yielding unique 3D microstructures.
2 Tourovskaia, A., Barber, T., Wickes, B., Hirdes, D., Grin, B., Castner, D. G., Healy, K. E., and Folch, A., "Micropatterns of Chemisorbed Cell Adhesion-Repellent Films Using Oxygen Plasma Etching and Elastomeric Masks", Langmuir 19, 4754 (2003).
This paper reports a method to confine cells for many days to micropatterns of proteins adsorbed on glass.
1 Folch, A. and Toner, M. "Microengineering of Cellular Interactions", Annual Rev. of Biomedical Engineering 2, 227 (2000).
This paper (cited over 500 times) is a review that covers microfabrication techniques used to modulate cell-substrate, cell-cell, and cell-medium interactions.


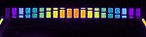
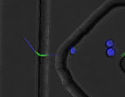
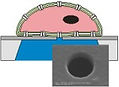
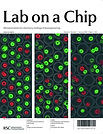

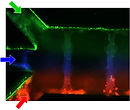
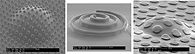

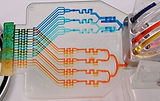

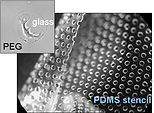
bottom of page